Phytochemical profiles and antimicrobial activity of Coleus lanuginosus (hochst. ex benth.) and Microglossa pyrifolia (Lam.) Kuntze extracts
Quetta Mbinya Mulilya, Erastus Mwaringa Mwadondo, Simeon Zachariah Mogaka, Robert Nesta Kagali, Sammy Indire Wanakai, Julia Akhwale Khayeli, Kenneth Omondi Ogila
Corresponding author: Quetta Mbinya Mulilya, Department of Zoology, College of Pure and Applied Sciences, Jomo Kenyatta University of Agriculture and Technology, Nairobi, Kenya
Received: 12 Mar 2024 - Accepted: 21 Aug 2024 - Published: 03 Sep 2024
Domain: Bacteriology, Microbiology, Infectious disease
Keywords: Antimicrobial activity, phytochemicals, Coleus lanuginosus, Microglossa pyrifolia
©Quetta Mbinya Mulilya et al. PAMJ-One Health (ISSN: 2707-2800). This is an Open Access article distributed under the terms of the Creative Commons Attribution International 4.0 License (https://creativecommons.org/licenses/by/4.0/), which permits unrestricted use, distribution, and reproduction in any medium, provided the original work is properly cited.
Cite this article: Quetta Mbinya Mulilya et al. Phytochemical profiles and antimicrobial activity of Coleus lanuginosus (hochst. ex benth.) and Microglossa pyrifolia (Lam.) Kuntze extracts. PAMJ-One Health. 2024;15:1. [doi: 10.11604/pamj-oh.2024.15.1.43247]
Available online at: https://www.one-health.panafrican-med-journal.com/content/article/15/1/full
Research 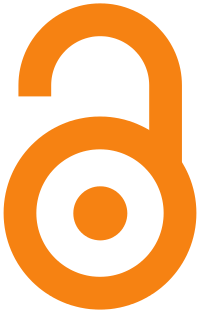
Phytochemical profiles and antimicrobial activity of Coleus lanuginosus (hochst. ex benth.) and Microglossa pyrifolia (Lam.) Kuntze extracts
Phytochemical profiles and antimicrobial activity of Coleus lanuginosus (hochst. ex benth.) and Microglossa pyrifolia (Lam.) Kuntze extracts
Quetta Mbinya Mulilya1,&, Erastus Mwaringa Mwadondo1,
Simeon Zachariah Mogaka1,
Robert Nesta Kagali1, Sammy Indire Wanakai2,
Julia Akhwale Khayeli1,
Kenneth Omondi Ogila1
&Corresponding author
Introduction: Coleus lanuginosus and Microglossa pyrifolia are woody perennial herbs that are widely cultivated in Africa, Asia, and Australia. The use of crude extracts from leaves, stems and roots of these plants has been reported in traditional folklore medicine as a remedy for varied diseases. This study sought to investigate the phytochemical composition and evaluate the antimicrobial activities of the stem and leaf extracts of Coleus lanuginosus and Microglossa pyrifolia.
Methods: the stem and leaf of both plants were collected from eastern Kenya, air-dried, and crushed into powder, and the crude extract was obtained using Soxhlet apparatus with aqueous and methanolic solvents. The antibacterial and antifungal activity was evaluated against gram-positive bacteria (Bacillus subtilis, Staphylococcus aureus) and gram-negative bacteria (Pseudomonas aeruginosa, Escherichia coli) and the fungus Candida albicans. Antibacterial and antifungal activity were evaluated by the disc diffusion method. The minimum inhibitory concentration (MIC) of the extracts was also determined.
Results: qualitative and quantitative phytochemical screening was done on the two plants to establish the presence of biologically important secondary metabolites. Both methanolic and aqueous crude extracts of C. lanuginosus and M. pyrifolia demonstrated to possess antibacterial and antifungal activity against the selected microbes. The extracts showed minimum inhibitory concentration (MIC) activity against Bacillus subtilis, Staphylococcus aureus Pseudomonas aeruginosa and Escherichia coli ranging from 6.25mg-50mg/kg with M. pyrifera leaf extract resulting in the highest zones of inhibition. The phytochemical constituents identified were flavonoids, alkaloids, glycosides, phenols, saponins, steroids, and terpenoids, with alkaloids, glycosides, saponins, and steroids being the most abundant. Gas chromatography-mass spectrometry (GC-MS) analysis led to the identification of fifty-nine compounds with all the extracts containing at least two or more long-chain unsaturated fatty acids which have been linked to antimicrobial activity.
Conclusion: in conclusion, C. lanuginosus and M. pyrifolia extracts have great potential as a source of novel antimicrobial and antifungal agents and possess a wide range of bioactive phytochemical compounds with the potential for ethnomedicinal use.
Since ancient times, plants have been a great source of medicinal substances for human use. Numerous contemporary medications have been derived from plant extracts, and many of these have become essential components of conventional medical treatments [1]. Most of the medications used in modern medicine today are derived from natural sources. For example, artemisinin, derived from Artemisia annua, is used to treat malaria [2], and paclitaxel, derived from the Pacific yew tree, is used in cancer treatment [3]. Infectious diseases are still the most common cause of death, causing about one-fourth of all deaths worldwide [4]. Infections caused by antibiotic-resistant microorganisms have become more common, making the situation worse [5]. This makes it urgent to develop new antimicrobial drugs to fight and stop the spread of these resistant bacteria [6]. Plants with advanced properties have been underutilized as possible new drug sources. This makes them a great place to start looking for effective new antimicrobial agents [7]. This study aims to evaluate the antibacterial properties and phytochemical composition of Coleus lanuginosus and Microglossa pyrifolia, plants from the Lamiaceae [8] and Asteraceae families, respectively [9]. Several species from Plectranthus genus (Lamiaceae family) have been shown to have ethnopharmacological benefits, such as, respiratory-related problems and gastrointestinal, because of their anti-inflammatory, antifungal and antibacterial properties [10]. Various plants from the Asteraceae family are traditionally used in folk medicine to treat a range of ailments, as documented by ethnomedicinal studies. These ailments include digestive problems, skin conditions, fever, jaundice, respiratory issues (such as asthma), hair loss and greying, and liver disorders [11]. The rationale for selecting these plants lies in their traditional use and reported medicinal properties, which justify further scientific investigation. Evaluating their antibacterial effects can provide empirical support for traditional claims and identify new therapeutic options.
Antimicrobial activity of extracts from C. lanuginosus and M. pyrifolia will be tested against both gram-positive (Bacillus subtilis ATCC 6633, Staphylococcus aureus ATCC 25923) and gram-negative bacteria (Pseudomonas aeruginosa ATCC 27853, Escherichia coli ATCC 25922) as well as a fungus Candida species. Gram-positive and gram-negative bacteria represent different classes of pathogens with distinct cell wall structures, thus providing a thorough assessment of the plants' antibacterial efficacy [12] Candida species was included due to their clinical relevance in opportunistic infections, particularly in immunocompromised patients [13]. Evaluating the effectiveness of C. lanuginosus and M. pyrifolia against these diverse microorganisms will help determine their potential as broad-spectrum antimicrobial agents. This research could benefit healthcare settings by offering new therapeutic options and potentially improve public health and socio-economic conditions by addressing infection-related challenges in communities and hospitals in Kenya.
Study design: a laboratory based experimental design was employed to examine the phytochemical composition and evaluate the antimicrobial activities of stem and leaf extracts of C. lanuginosus and M. pyrifolia.
Setting: this study was conducted at the Government of Kenya (GOK) Botany, GOK Chemistry and Chromatography and Mass spectrometry laboratories in Jomo Kenyatta University of Agriculture and Technology (JKUAT).
Plant collection: leaves and stem samples of M. pyrifera and C. lanuginosus were collected from Mbooni, Kenya (2.2559°S, 37.8937° E; about 182 km from Nairobi) in November 2022, cleaned, air-dried, and then processed into fine powder for extraction. The plants were verified by the Jomo Kenyatta University of Agriculture and Technology (JKUAT) Botany Herbarium (REF: QM-JKUATBH/001/2023 and QM-JKUATBH/002/ABC-2023) respectively and voucher specimens with their corresponding identification codes were kept in the Botany Department of the National Museum of Kenya in Nairobi for future use.
Plant materials extraction: plant materials were extracted using aqueous and methanolic solvents. Briefly, 400 grams of powdered leaves and stem from both plants were mixed with 900 ml of distilled water and boiled for 20 minutes [14]. After cooling, the supernatant was separated, centrifuged and filtered. The filtrates were frozen at -20°C and then dried in a freeze-drier. The extracts were weighed and kept at 4°C. Methods by Harborne (1976) and adjusted by Afolayan et al. [15] were used to determine the percentage yields of extracts.
Preliminary phytochemical and quantitative phytochemical screening: the techniques employed to test for the presence of saponins, terpenoids, tannins, steroids, glycosides, flavonoids, alkaloids and phenols were done following standard procedures by Shaikh et al. [16] and Bashir et al. [17]. Quantitative Phytochemical Screening was done as described by Khanal and Garg P et al. [18,19].
Determination of total saponins: plant extract was mixed with ethanol, heated, filtered, and double extracted. The extracts were combined and quartered over a water bath. In a separating funnel, 20cm3 of dithyl ether was added, agitated, and recovered. The sample was purified in an oven with 60cm3 of n-butanol [20].
Determination of total tannins: plant samples (0.1 g) were subjected to methanol extraction, mixed with standards, and evaluated using the Folin–Ciocalteu method with a standard of garlic acid. After adding reagents and a 40-minute standing period, absorbance was then measured using an Ultra-violet visible (UV-VIS) spectrophotometer.
Determination of total flavonoids: a Rutin equivalent (RE)/g dry weight scale, was used to calculate the flavonoid concentration after standards were chemically treated, following a calibration curve.
Determination of total alkaloids: using 10% acetic acid in ethanol, the sample was combined for four hours. An ammonium solution was added to the concentrated extract, resulting in the formation of a white precipitate. After washing and drying, the residue was evaluated for alkaloid percentage [21].
Determination of total phenols: plant extracts phenolic content was measured via the Folin-Ciocalteau method using 80% methanol extraction. Phenolics were measured using spectrophotometry at 769 nm and expressed as milligrams of equivalent garlic acid per gram of dry extract.
Phytochemical profiling of C. lanuginosus and M. pyrifera stem and leaf Methanolic extract: with a few adjustments, the plant extracts were analyzed using gas chromatography-mass spectrometry (GC-MS) as described by Olivia NU et al. [22]. One (1) mL of methanol was dissolved with 100 mg of sample. The mixture underwent a 10-second vortex, a 10-minute sonication, and a 5-minute 14,000 rpm centrifugation. To prepare 100 ng/μL, the supernatant was separated and diluted. Three replicates were used for the analysis. The mass spectrometer differentiated the metabolites, which were used to quantify the compounds. Using retention durations and mass spectra comparable to standards (where available) along with the National Institute of Standards and Technology (NSIT) database, the found compounds were identified using the Automated Mass Spectral Deconvolution and Identification System (AMDIS) program. To identify the discovered chemicals, comparison with the spectra of known compounds from the literature was also performed.
Test organisms: the selected bacterial strains for this study were gram-positive bacteria Bacillus subtilis American Type Culture Collection (ATCC 6633), Staphylococcus aureus (ATCC 25923) and gram-negative bacteria Pseudomonas aeruginosa (ATCC 27853), Escherichia coli (ATCC 25922) and the fungus Candida albicans (ATCC 90028). The organisms were from a culture collection kept at the Government of Kenya (GOK) Laboratory, Department of Botany, Jomo Kenyatta University of Agriculture and Technology. The bacteria were cultured on nutrition agar and kept on nutrient agar slants in order to determine their purity.
Preparation of inocula: to create active cultures for the tests, a loopful of cells was taken from the stock cultures maintained on nutrient agar slopes and placed in test tubes containing Mueller-Hinton broth (MHB) for bacteria and Sabouraud dextrose broth (SDB) for fungi and cultured overnight at 37°C. After incubation, cultures were diluted with MHB and SDB to match the McFarland turbidity standard of 2x106 colony-forming unit for bacteria and 2x107 spores/ml for fungal strain [23].
Antibacterial activity of the extracts: this was accomplished using the disk diffusion technique, outlined by [24]. Sterile filter paper discs (Whatman No.1) each measuring 5 millimeters in diameter, were soaked with various plant extracts of different concentrations, 1% dimethylsulfoxide (negative control), Rifampin 5mg/ml, and Gentamycin (positive controls). To prepare the inoculum, a broth culture was diluted to match a 0.5 McFarland turbidity standard and incubated overnight. A sterile wire loop was used to collect a broth culture of a particular bacteria, which was then streaked across the surface of Mueller-agar plate to create a bacterial lawn using an aseptic technique. The disks that had been soaked with the various plant extracts, negative and positive controls, were placed on the solid agar medium with forceps. The experiments were done in triplicates and the plates were incubated at 37°C for 18-24 hours. After incubation, the zones of inhibition were measured in millimeters using a transparent ruler and noted.
Evaluation of the minimum inhibitory concentration (MIC) of the extracts: this was done by broth microdilution method as described by Kebede et al. [25]. To prepare a standard inoculum, a small sample of colonies was collected using a wire loop and added tryptic soy broth. This broth was incubated at 35-37°C until the bacteria grew to a cloudiness comparable to or higher than a reference standard known as 0.5 McFarland. To match the turbidity of the McFarland 0.5 standard, the culture was adjusted with sterile distilled water. The absorbance was then measured using a Spectrophotometer.
Antifungal activity of the extracts: this was done as described by Falana et al. [27] with a few modifications. Preparation of the culture medium was carried out as directed by the manufacturer. After the sterilized medium was cooled to around 50°C and exactly 20 ml was dispensed into sterile petri dishes and allowed to solidify. Petri dishes were inoculated aseptically with Candida albicans (ATCC 90028), and subsequently incubated 31°C for 24 hours. Using a sterile wire loop, the well-isolated Candida albicans colonies were picked and suspended in sterile 0.9% normal saline. The inoculum's turbidity was compared to the McFarland. To achieve a concentration of 2 x 107 cells/ml, 74 ml of sterile medium and 1 ml of microbial suspension in normal saline were mixed and maintained at 45°C. Approximately 1 ml of the fungus strain was added to sterilized plates 15 milliliters of sterile Sabouraud dextrose agar (SDA) medium were added to each plate containing 1 milliliter of the fungal strain and mixed homogeneously. Disks which had been soaked with the various plant extracts, and fluconazole (positive control) were placed on the solid agar medium with a forceps. The plates were incubated for 24 hours at 35 to 37°C. After incubation, the zones of inhibition were measured in millimeters using a transparent ruler and noted.
Statistical data analysis: raw data was captured in a data book, transferred to a Microsoft Excel spreadsheet, and then exported to the statistical analysis program SPSS version 27.0. Descriptive statistics were expressed as mean ± standard deviation. One-way analysis of variance was used to determine the statistical difference among different treatment groups followed by Bonferroni post hoc test for comparison of means between different treatment groups. The level of significance was set at 95% (p ≤ 0.05).
Percentage yields of C. lanuginosus and M. pyrifera extracted: the aqueous extracts of C. lanuginosus and M. pyrifera leaves recorded yields ranging from 23% to 18% and 11% to 7% for the methanolic extracts respectively (Figure 1).
Preliminary phytochemical screening of extracts: the preliminary phytochemical screening of aqueous and methanolic leaf and stem extracts of C. lanuginosus and M. pyrifera revealed a range of bioactive compounds such as; saponins, terpenoids, tannins, steroids, glycosides flavonoids, alkaloids and phenols as shown in Table 1.
Quantitative phytochemical analysis: the quantitative phytochemical estimation revealed that C. lanuginosus and M. pyrifera leaf and stem powders contained a significant amount of saponin, tannins, flavonoids, alkaloid, and phenolic content. The saponin content was estimated quantitatively and was found to range between 2.1 ± 0.2 to 1.12 ± 0.08 mg/l in different plant parts from the two plants but the maximum content was observed in M. pyrifera leaf powder 2.1 ± 0.2 mg/l. Likewise, all the plant sample powders showed a significant amount of flavonoids, tannins, alkaloids, and phenols, starting from 2.37 ± 0.003 mg/l ,0.079 ± 0.001 mg/l, 1.0 ± 0.2 mg/l and 0.297 ± 0.000 mg/l, respectively as shown in Table 2.
Phytochemical profile of methanolic M. pyrifera leaf and stem extracts: gas chromatography-mass spectrometry analysis of leaf and stem extracts of M. pyrifera showed Sesquiterpenes (Iso-sativene, Beta-copaene, Alpha-ylangene Caryophyllene), alcohols (Trans-Geranylgeraniol and Phytol), terpenes (1-Isopropyl-4, 7-dimethyl-1, 2, 3, 5, 6, 8a hexahydronaphthalene,1, 1, 7, 7a-Tetramethyl-1a, 2, 6, 7, 7a, 7b-hexahydro-1H-cyclopropa (a) naphthalene) and a triterpenoid (Lupeol, trifluoroacetate). Beta-copaene was the most abundant while Alpha-ylangene was the least. (Figure 2, Figure 3, Annex 1).
Phytochemical profile of methanolic C. lanuginosus leaf and stem extracts: gas chromatography-mass spectrometry analysis of leaf and stem extracts of C. lanuginosus showed sesquiterpenes (Germacrene D, beta. Caryophyllene, and Beta-Vatirenene), monoterpenoids (Camphor, Carveol) alkynes (Undecane, 3-Butyn-1-ol) and Fatty acids (Pentadecanoic acid, 9-Octadecenoic acid, Hexadecatrienoic acid and tridecanoic (Figure 4, Figure 5, Annex 2).
Antimicrobial activities of methanol extracts of C. lanuginosus and M. pyrifera on selected test organisms: the methanolic extracts revealed antibacterial activity against E. coli, B. subtilis, P. aeruginosa, and S. aureus at concentrations of 6.25, 12.5, 25, and 50 mg/ml. This was shown by clear areas (zones of inhibition) that were more than 5 mm wide in diameter after the impregnation of paper discs. When tested against various bacterial strains the negative control dimethyl sulfoxide (DMSO) didn´t show any antibacterial activity (Table 3, Table 4). The reference drugs, rifampin, and gentamycin, had significantly stronger antibacterial effects compared to the MeOH extracts at all the concentrations tested against. S. aureus, P. aeruginosa, B. subtilis, and E. coli (Table 3, Table 4). The antibacterial activity of MeOH extracts at the concentrations of 6.25, 12.5, 25, and 50mg/ml exhibited significantly greater antibacterial activity against E. coli, B. subtilis, P. aeruginosa, and S. aureus (Table 3, Table 4). Methanol extracts at 50 mg/ml showed significantly higher antimicrobial activity against all tested bacteria strains compared to lower concentrations (6.25, 12.5, and 25 mg/ml). (Table 3, Table 4). Similarly, the antibacterial effect of the MeOH extracts at the concentrations of 50 and 25 mg/ml was statistically higher than those of 6.25 and 12.5 mg/ml against all the tested strains (Table 3, Table 4). Based on these results, all the methanolic extracts showed broad-spectrum antibacterial activities particularly at higher concentrations Both C. lanuginosus and M. pyrifolia methanolic extracts showed some antifungal efficacy against Candida albicans. The antifungal effects appeared to be influenced by both the specific plant extracts and their concentrations. Increased antifungal effects were shown with C. lanuginosus methanolic stem and M. pyrifolia methanolic stem at lower concentrations, indicating a concentration-dependent trend. The activity of M. pyrifolia methanolic leaf and C. lanuginosus methanolic leaf (Table 3, Table 4), was more consistent throughout concentrations. Maximum inhibition was shown by fluconazole, a positive control, demonstrating its efficacy against fungi. This variation highlights the relationship between fungi and plant compounds. The results highlight the significance of determining the best doses for maximizing antifungal effectiveness, a factor that could differ between plant extracts. These findings show that these plant extracts have a lot of potential as natural antifungal agents and call for more research into their processes, potential interactions, and safety profiles. These extracts might be useful for developing antifungal treatments or serving as the basis for further study.
Antimicrobial activities of aqueous extracts of C. lanuginosus and M. pyrifera on selected bacterial strains: for the aqueous extracts, the best results were observed against E. coli and S. aureus at higher concentrations. The negative control had a similar effect to that of aqueous M. pyrifera stem extract at all tested concentrations against P. aeruginosa and S. aureus (Table 5). The stem extract of C. lanuginosus demonstrated no antibacterial effect at all concentrations tested against B. subtilis while the leaf extract of C. lanuginosus demonstrated no antibacterial effect at all concentrations tested against E. coli (Table 6). The concentrations of aqueous extracts that lacked antibacterial activity never had zones with a diameter of 5 mm (Table 5, Table 6). The antibacterial effects of rifampin and gentamycin, the reference drugs, were significantly stronger compared to the aqueous extracts at all concentrations tested against S. aureus, P. aeruginosa, B. subtilis, and E. coli (Table 6). The antibacterial activity of plant extracts was dose-dependent only when tested against specific bacteria. At concentrations of 50 and 25 mg/ml, both M. pyrifera and C. lanuginosus extracts had significantly greater inhibitory effects against E. coli, B. subtilis, and S. aureus compared to lower concentrations (12.5 and 6.25 mg/ml). The C. lanuginosus extract also showed significantly higher inhibitory effects against P. aeruginosa at these concentrations. (Table 5, p < 0.05) (Table 6). Aqueous extracts generally demonstrated the best antifungal activity at higher doses, notably at 50 mg/ml, (Table 5, Table 6) as seen by the highest inhibition values. In contrast, decreased concentrations of these extracts showed a reduction in antifungal activity. Notably, M. pyrifera aqueous stem extract maintained its antifungal activity constant across all concentrations. Fluconazole exhibited considerable inhibition, demonstrating its potent antifungal effects. In general, these findings point out concentration-dependent effects of the extracts' antifungal activity and suggest prospective uses as natural antifungal drugs, pending further research into their processes and safety profiles.
Antibacterial activities of minimum inhibition concentrations (MIC) of aqueous and methanolic extracts: the lowest concentration of the extracts that completely inhibited the microorganism´s growth was taken to be MIC. M. pyrifera aqueous leaf extracts were active against all the four bacteria with P. aeruginosa having lowest MIC which indicated that it was sensitive to the extracts since the inhibition occurred at lower concentrations conversely, E. coli had the highest MIC (Table 7) p-value of 0.032, indicating statistical significance, the leaf extracts showed broad spectrum antimicrobial activity. The M. pyrifera aqueous stem extracts also revealed some activity against all the bacteria tested, with P. aeruginosa having lowest MIC and E. coli the highest MIC. The aqueous leaf extract of C. lanuginosus showed similar activity to the aqueous stem extract of M. pyrifera in inhibiting bacterial growth (Table 7). However, the stem extracts required higher concentrations to inhibit the growth of the four bacteria, indicating that they were less effective than the leaf extract (Table 7). Statistical analysis revealed a significant difference between the two extracts, with an exact p-value of 0.009, suggesting that the leaf extract was more effective.
On the other hand, M. pyrifera leaf methanolic extract had MIC ranging from 6.25 to 50 mg/ml for E. coli, B. subtilis P. aeruginosa and S. aureus. The extract was active against all the tested organisms at all tested concentrations (Table 7). Leaf extracts from the plant C. lanuginosus effectively inhibited the growth of four different bacteria. The most sensitive bacterium was Pseudomonas aeruginosa, which exhibited growth inhibition at low extract concentrations. On the other hand, E. coli was the bacterium with the least sensitivity; it needed larger concentrations of extracts to prevent its growth. The leaf extracts showed broad spectrum antimicrobial activity. Methanolic extracts from the stems of C. lanuginosus showed activity against all the test bacteria, with the lowest MIC against P. aeruginosa and the highest MIC against B. subtilis (Table 7).
The extracts of C. lanuginosus and M. pyrifera possessed important phytochemical compounds, tannins, steroids, glycosides alkaloids but not saponins, terpenoids, flavanoids, and phenols which are known for their diverse biological properties. All the methanolic extracts of C. lanuginosus and M. pyrifera showed antibacterial effects against all the different microorganisms that were tested. This highlights their broad-spectrum antibacterial properties, by affecting bacteria that are both gram-positive and gram-negative. Although the aqueous extracts did not inhibit all of the tested microorganisms, the antibacterial activities that were observed could have been due to the presence of secondary metabolites such as alkaloids, tannins, saponins, and steroids which have been linked to antimicrobial properties [27]. The antibacterial activity of the methanolic extracts could be due to the presence of various phytochemicals such as; alkaloids, flavonoids, steroids, saponins, terpenoids, and glycosides [28]. Even though the aqueous leaf and stem extracts of C. lanuginosus lacked some of the phytochemicals found in the other extracts, surprisingly, these extracts still exhibited significant antibacterial properties which can be attributed to the presence of tannins, steroids, glycosides and alkaloids [29]. The quantitative analysis of leaf and stem powders of C. lanuginosus and M. pyrifera showed high amounts of tannins in both plant parts. This observation is consistent with previous research, which reported that mangrove species showed tannins contained in bark and stems were higher (66.6%) than those in leaves (33.4%) [30]. In contrast, our research found that in C. lanuginosus, stems contained 50.89% tannins while leaves contained 37.57%, and in M. pyrifolia, stems contained 49.11% tannins while leaves contained 37.57% tannins.
Alkaloids are commonly recognized for their potent antimicrobial properties [31]. Flavonoids play significant roles in plant biochemistry and physiology they act as antioxidants, protecting cells from damage, inhibit enzymes, regulating various biological processes, they also act as precursors for toxic substances, affecting plant defence and interactions with other organisms [32]. Flavonoids possess antimicrobial and fungicidal properties, contributing to plant disease resistance [33]. Numerous studies have demonstrated the antioxidant effects of various phenolic compound-rich phenolic portions of diverse medicinal plants [34]. Phenols, found in various plants, play crucial roles in nutrient absorption, protein production, enzyme functions, and photosynthesis. They also serve as structural elements and contribute to the defense mechanisms of plants against organisms [35]. Terpenoids have antimicrobial properties. They can damage cell membranes, making them more permeable. This can prevent the release of prostaglandins, which are inflammatory molecules [36]. According to reports, saponins are known to be an effective expectorant. This property, explains why the plant C. lanuginosus has been traditionally employed to treat respiratory infections [37]. Compounds like tannins and steroids found in medicinal plants possess anti-inflammatory and antibacterial properties [38].
Gas chromatography mass spectrometry revealed 59 compounds in stem and leave methanolic extract of C. lanuginosus and M. pyrifera. Among the identified compounds, Tridecanoic acid a fatty acid that has been reported to have antimicrobial activity [39]. Fatty acids with multiple unsaturated bonds, like Pentadecanoic and Hexadecatrienoic acids, have the ability to kill harmful bacteria like the highly resistant Staphylococccus aureus, Helicobacter pylori, and the fungus Candida albicans [40]. Sesquiterpenes such as Beta-copaene have strong antimicrobial activities making them effective for both bacteria and fungi [41]. Caryophyllene possesses qualities that alleviate pain and reduce inflammation. These effects arise from its interaction with the body's endocannabinoid system, which is also targeted by cannabis [42]. Terpenes possess diverse medicinal properties, such as antifungal capabilities that combat fungal infections, anti-inflammatory abilities that reduce inflammation, and anticancer properties that may prevent or fight cancer [43].
Plant extracts derived from M. pyrifera and C. lanuginosus showed strong antibacterial activity against both Gram-negative (P. aeruginosa and E. coli) and Gram-positive (B. subtilis and S. aureus) bacteria. They also effectively inhibited the growth of the fungus C. albicans. When analysed by GC-MS, methanolic extracts from M. pyrifera and C. lanuginosus contained bioactive compounds with potential applications in treating diverse infections. In summary, both extracts show significant promise as sources of new antimicrobial and antifungal agents. They contain a diverse array of bioactive plant chemicals that could be used in traditional medicine. Further research is recommended to explore the inhibition activity of these plant extracts against drug-resistant bacteria. Investigating their effectiveness against resistant strains could provide valuable insights and contribute to the development of new antimicrobial agents to combat the growing issue of antibiotic resistance.
What is known about this topic
- Both aqueous and methanolic extracts of M. pyrifera and C. lanuginosus demonstrated antimicrobial activity against the tested bacterial strains with the methanolic extracts being more effective in inhibiting bacterial growth than aqueous extracts;
- The antibacterial activities of the plants could be due to the presence of important phytochemical compounds in the extracts;
- M. pyrifera and C. lanuginosus plants revealed the presence of biologically important compounds which have been linked to antimicrobial activity.
What this study adds
- This research study significantly supports the claims made by traditional herbal practitioners, who have long believed that these plants possess medicinal properties that can aid in the treatment and prevention of infectious diseases;
- The antimicrobial properties found in these two plants have potential applications in treating infectious diseases caused by the microorganisms mentioned.
The authors declare no competing interests.
All authors played an equivalent role and made substantial contributions to the completion of this work. All the authors have read and agreed to the final manuscript.
We greatly appreciate the contributions of the Technologists in the Technology Department of Jomo Kenyatta University of Agriculture and Technology (JKUAT) and those working in the Government of Kenya Biology Laboratory located at JKUAT for their indispensable assistance in conducting our experiments.
Table 1: preliminary qualitative phytochemical analysis of aqueous and methanolic extracts C. lanuginosus and M. pyrifera leaves and stems
Table 2: quantitative phytochemical analysis of C. lanuginosus and M. pyrifera leaf and stem powders
Table 3: antimicrobial effect of MeOH extracts of C. lanuginosus against E. coli, B. subtilis, P. aeruginosa, S. aureus and C.albicans
Table 4: antimicrobial effect of MeOH extracts of M. pyrifera against E. coli, B. subtilis, P. aeruginosa, S. aureus and C. albicans
Table 5: antimicrobial effect of aqueous extracts of M. pyrifera against E. coli, B. subtilis, P. aeruginosa, S. aureus and C.albicans
Table 6: antimicrobial effect of aqueous extracts of C. lanuginosus against E. coli, B. subtilis, P. aeruginosa, S. aureus and C. albicans
Table 7: minimum Inhibitory concentrations of aqueous extracts and methanolic extracts of M. pyrifera and C. lanuginosus against test microbes
Figure 1: percentage yields of C. lanuginosus and M. pyrifera extract
Figure 2: GC-MS chromatogram of M. pyrifera leaf extract
Figure 3: GC-MS chromatogram of M. pyrifera stem extract
Figure 4: GC-MS chromatogram of C. lanuginosus leaf extract
Figure 5: GC-MS chromatogram of C. lanuginosus stem extract
Annex 1: compounds detected in both MeOH leaf and stem extracts of M. pyrifera through GC-MS (PDF - 72 KB)
Annex 2: compounds detected in both MeOH leaf and stem extracts of C. lanuginosus through GC-MS (PDF - 85 KB)
- Chia TY, Gan CY, Murugaiyah V, Hashmi SF, Fatima T, Ibrahim L et al. A narrative review on the phytochemistry, pharmacology and therapeutic potentials of clinacanthus nutans (Burm. f.) lindau leaves as an alternative source of future medicine. Molecules. 2021 Dec 27;27(1):139. PubMed | Google Scholar
- Ekiert H, Świątkowska J, Klin P, Rzepiela A, Szopa A. Artemisia annua, Importance in Traditional Medicine and Current State of Knowledge on the Chemistry, Biological Activity and Possible Applications. Planta Med. 2021 Jul;87(8):584-599. PubMed | Google Scholar
- Yan-Hua YA, Jia-Wang MA, Xiao-Li TA. Research progress on the source, production, and anti-cancer mechanisms of paclitaxel. Chinese Journal of Natural Medicines. Chin J Nat Med. 2020 Dec;18(12):890-897. PubMed | Google Scholar
- Frenkel LD. Infectious diseases as a cause of global childhood mortality and morbidity: Progress in recognition, prevention, and treatment. Adv Pediatr Res. 2018 Apr 10; 5(14):1-11. Google Scholar
- Süntar I. Importance of ethnopharmacological studies in drug discovery: role of medicinal plants. Phytochemistry Reviews. 2020 Oct;19(5):1199-209. Google Scholar
- Keshamma E, Paswan SK, Kumar R, Saha P, Trivedi U, Chourasia A et al. Alkaloid Based Chemical Constituents of Ocimum santum & Cinchona Bark: A Meta Analysis. Journal for Research in Applied Sciences and Biotechnology. 2022 Jun 30;1(2):35-42. Google Scholar
- MacNeil A, Glaziou P, Sismanidis C, Date A, Maloney S, Floyd K. Global epidemiology of tuberculosis and progress toward meeting global targets-worldwide 2018. MMWR Morb Mortal Wkly Rep. 2020 Mar 20;69(11):281-285. PubMed | Google Scholar
- Al-Juhani WS, Khalik KN. Identification and molecular study of medicinal Plectranthus species (Lamiaceae) from Saudi Arabia using plastid DNA regions and ITS2 of the nrDNA gene. Journal of King Saud University-Science. 2021 Jul 1;33(5):101452. Google Scholar
- Timothy O. Plants used in antivenom therapy in rural Kenya: ethnobotany and future perspectives. J Toxicol. 2020 Jun 16;2020:1828521. PubMed | Google Scholar
- Antão, AR, Bangay G, Domínguez-Martín EM, Díaz-Lanza AM, Ríjo P. Plectranthus ecklonii benth: a comprehensive review into its phytochemistry and exerted biological activities. Front Pharmacol. 2021 Nov 30;12:768268. PubMed | Google Scholar
- Chagas-Paula DA, Oliveira RB, Rocha BA, Da Costa FB. Ethnobotany, chemistry, and biological activities of the genus Tithonia (Asteraceae). Chem Biodivers. 2012 Feb;9(2):210-35. PubMed | Google Scholar
- Tavares TD, Antunes JC, Padrão J, Ribeiro AI, Zille A, Amorim MT et al. Activity of specialized biomolecules against gram-positive and gram-negative bacteria. Antibiotics (Basel). 2020 Jun 9;9(6):314. PubMed | Google Scholar
- Ciurea CN, Kosovski IB, Mare AD, Toma F, Pintea-Simon IA, Man A. Candida and candidiasis-opportunism versus pathogenicity: a review of the virulence traits. Microorganisms. 2020 Jun 6;8(6):857. PubMed | Google Scholar
- Letaief T, Garzoli S, Laghezza Masci V, Mejri J, Abderrabba M, Tiezzi A et al. Chemical composition and biological activities of tunisian Ziziphus lotus extracts: Evaluation of drying effect, solvent extraction, and extracted plant parts. Plants (Basel). 2021 Dec 2;10(12):2651. PubMed | Google Scholar
- Afolayan M, Srivedavyasasri R, Asekun OT, Familoni OB, Orishadipe A, Zulfiqar F et al. Phytochemical study of Piliostigma thonningii, a medicinal plant grown in Nigeria. Med Chem Res. 2018 Oct;27(10):2325-2330. PubMed | Google Scholar
- Shaikh JR, Patil M. Qualitative tests for preliminary phytochemical screening: An overview. International Journal of Chemical Studies. 2020 Mar;8(2):603-6088. Google Scholar
- Bashir SF, Kumar G. Preliminary phytochemical screening and in vitro antibacterial activity of Plumbago indica (Laal chitrak) root extracts against drug-resistant Escherichia coli and Klebsiella pneumoniae. Open Agriculture. 2021 Jun 28;6(1):435-444. Google Scholar
- Khanal S. Qualitative and quantitative phytochemical screening of Azadirachta indica Juss. Plant parts. 2021;122-127. PubMed | Google Scholar
- Garg P, Garg R. Phytochemical screening and quantitative estimation of total flavonoids of Ocimum sanctum in different solvent extract. Pharma Innov J. 2019;8(2):16-21. Google Scholar
- Le Bot M, Thibault J, Pottier Q, Boisard S, Guilet D. An accurate, cost-effective and simple colorimetric method for the quantification of total triterpenoid and steroidal saponins from plant materials. Food Chem. 2022 Jul 30;383:132597. PubMed | Google Scholar
- Pu ZH, Dai M, Xiong L, Peng C. Total alkaloids from the rhizomes of Ligusticum striatum: a review of chemical analysis and pharmacological activities. Nat Prod Res. 2022 Jul;36(13):3489-3506. PubMed | Google Scholar
- Olivia NU, Goodness UC, Obinna OM. Phytochemical profiling and GC-MS analysis of aqueous methanol fraction of Hibiscus asper leaves. Future Journal of Pharmaceutical Sciences. 2021 Dec;7:1-5. Google Scholar
- Sutton SV, Geis PA. Antimicrobial preservative efficacy and microbial content testing. InCosmetic microbiology. CRC Press. 2020 Dec 6;67-93. Google Scholar
- Schön T, Werngren J, Machado D, Borroni E, Wijkander M, Lina G et al. Antimicrobial susceptibility testing of Mycobacterium tuberculosis complex isolates-the EUCAST broth microdilution reference method for MIC determination. Clin Microbiol Infect. 2020 Nov;26(11):1488-1492. PubMed | Google Scholar
- Kebede B, Shibeshi W. In vitro antibacterial and antifungal activities of extracts and fractions of leaves of Ricinus communis Linn against selected pathogens. Vet Med Sci. 2022 Jul;8(4):1802-1815 PubMed | Google Scholar
- Hassan A, Ullah H. Antibacterial and antifungal activities of the medicinal plant veronica biloba. Journal of chemistry. 2019;2019(1):5264943. Google Scholar
- Falana MB, Nurudeen QO. Evaluation of phytochemical constituents and in vitro antimicrobial activities of leaves extracts of Calotropis procera against certain human pathogens. Notulae Scientia Biologicae. 2020 Jun 29;12(2):208-221. Google Scholar
- Zulkifli L, Basri MH, Syukur A. Antibacterial activity of Vitex trifolia methanol extract against pathogenic bacteria. In: Journal of Physics: Conference Series. 2021 Apr 1;1869(1):012060. Google Scholar
- Mulatu G. Antibacterial activities of Calpurnia aurea against selected animal pathogenic bacterial strains. Adv Pharmacol Pharm Sci. 2020 Nov 17;2020:8840468. PubMed | Google Scholar
- Hilmi E, Sari LK, Siregar AS, Sulistyo I, Mahdiana A, Junaedi T et al. Tannins in mangrove plants in segara anakan lagoon, central java, indonesia. Biodiversitas Journal of Biological Diversity. 2021 Aug 9;22(8). Google Scholar
- Yan Y, Li X, Zhang C, Lv L, Gao B, Li M. Research progress on antibacterial activities and mechanisms of natural alkaloids: A review. Antibiotics (Basel). 2021 Mar 19;10(3):318. PubMed | Google Scholar
- Ullah A, Munir S, Badshah SL, Khan N, Ghani L, Poulson BG et al. Important flavonoids and their role as a therapeutic agent. Molecules. 2020 Nov 11;25(22):5243. PubMed | Google Scholar
- Biharee A, Sharma A, Kumar A, Jaitak V. Antimicrobial flavonoids as a potential substitute for overcoming antimicrobial resistance. Fitoterapia. 2020 Oct;146:104720. PubMed | Google Scholar
- Rahman MM, Rahaman MS, Islam MR, Rahman F, Mithi FM, Alqahtani T et al. Role of phenolic compounds in human disease: current knowledge and future prospects. Molecules. 2021 Dec 30;27(1):233. PubMed | Google Scholar
- Wagay NA, Lone R, Rafiq S, Bashir SU. Phenolics: a game changer in the life cycle of plants. Plant Phenolics in Sustainable Agriculture: 2020;1:241-275. Google Scholar
- Masyita A, Sari RM, Astuti AD, Yasir B, Rumata NR, Emran TB, Nainu F, Simal-Gandara J. Terpenes and terpenoids as main bioactive compounds of essential oils, their roles in human health and potential application as natural food preservatives. Food Chem X. 2022 Jan 19;13:100217. PubMed | Google Scholar
- Tatli Cankaya II, Somuncuoglu EI. Potential and prophylactic use of plants containing saponin-type compounds as antibiofilm agents against respiratory tract infections. Evid Based Complement Alternat Med. 2021 Jul 23;2021:6814215. PubMed | Google Scholar
- Osei Akoto C, Acheampong A, Boakye YD, Asante B, Ohene S, Amankwah F. Anthelminthic, anti-inflammatory, antioxidant, and antimicrobial activities and FTIR analyses of Vernonia camporum stem-bark. Journal of Chemistry. 2021 Sep 25;2021:1-15. Google Scholar
- Chowdhury SK, Dutta T, Chattopadhyay AP, Ghosh NN, Chowdhury S, Mandal V. Isolation of antimicrobial Tridecanoic acid from Bacillus sp. LBF-01 and its potentialization through silver nanoparticles synthesis: a combined experimental and theoretical studies. Journal of Nanostructure in Chemistry. 2021 Sep 25;2021:1-15. Google Scholar
- Cepas V, Gutiérrez-Del-Río I, López Y, Redondo-Blanco S, Gabasa Y, Iglesias MJ et al. Microalgae and cyanobacteria strains as producers of lipids with antibacterial and antibiofilm activity. Mar Drugs. 2021 Nov 27;19(12):675. PubMed | Google Scholar
- Al-Rekaby LS. Antibacterial activities for root extracts of (goldenrods) Solidago canadensis L treated by Nano and bio fertilizer. Al-Qadisiyah Journal of Pure Science. 2020 Nov 5; 25(4):1-14. Google Scholar
- Hashiesh HM, Sharma C, Goyal SN, Sadek B, Jha NK, Al Kaabi J et al. A focused review on CB2 receptor-selective pharmacological properties and therapeutic potential of ?-caryophyllene, a dietary cannabinoid. Biomed Pharmacother. 2021 Aug;140:111639. PubMed | Google Scholar
- Mabou FD, Yossa IB. TERPENES: Structural classification and biological activities. IOSR J Pharm Biol Sci. 2021;16:25-40. Google Scholar